Research
The Limburg Lab is interested broadly in supramolecular chemistry and photochemistry. Below you can find some current projects. Interested in joining us? Please send an email to Bart with a motivation letter and cv.
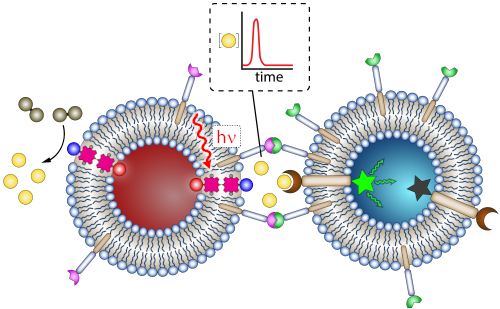
Synthetic Neurons and Artificial Photoactivated Synapses
The brain is a complex network of inter-connected neurons that communicate through synapses. The ERC-StG Project SYNAPS aims to for the first time mimic such synapses using liposomes as artificial cells, and visible light to trigger a signal from a ‘sender’- to a ‘receiver’-liposome. SYNAPS will maintain chemical isolation between liposome interiors, ensure local, time-bound communication between supramolecularly connected liposomes, and use light as an external stimulus and fuel. These concepts are essential to construct artificial tissues that can communicate on an individual liposome-to-liposome basis. In detail, a messenger compound will be locally photosynthesised through transmembrane electron transfer. The liposomes will be organised into an artificial synaptic cleft through the use of synthetic complementary clustering compounds. The messenger compound will be recognised by reversible and selective membrane-spanning receptors in the receiver liposome, that will output the signal through fluorescence. In addition, a reaction cascade network will be constructed involving the messenger to produce an artificial action potential, ensuring a time-bound dissipative signal. Altogether, SYNAPS will provide advances in systems chemistry by providing a nanoscale platform for communication between chemically isolated systems that resemble a soft-matter chemical computer, but also results that are useful for applications such as light-to-chemical energy conversion, chemical sensing and smart drug-delivery.
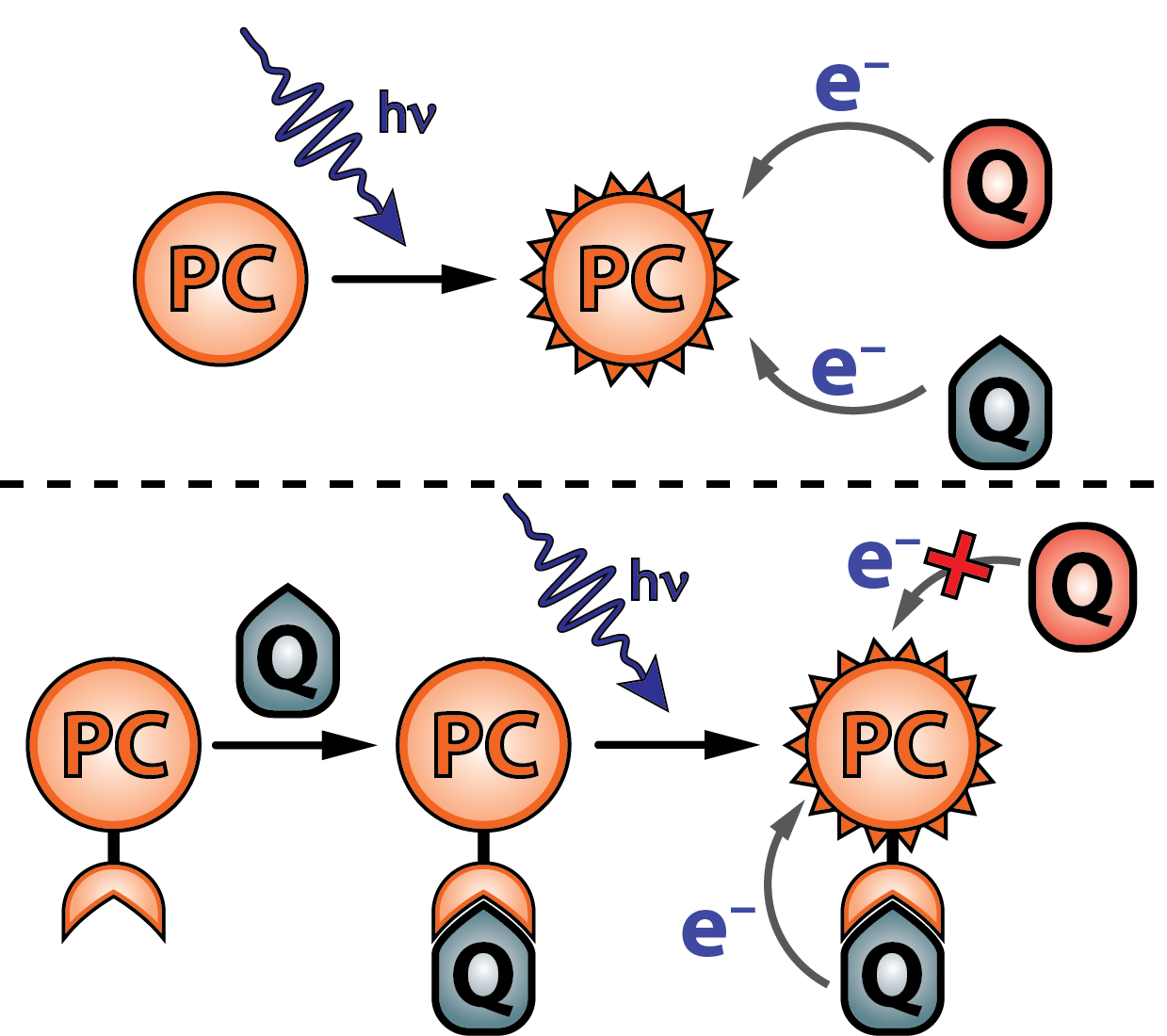
Photocatalyst Design for Improved Quantum Yields
Photoredox reactions generally suffer from low quantum yields. This is due to the thermodynamically highly-favored recombination of charges after initial photoinduced electron transfer. In addition, photoredox catalysis in organic synthesis suffers from low chemoselectivity which hinders a scope with highly electron-rich or electron-poor functional groups due to their reactivity with the excited state of the photocatalyst. In order to meet these challenges, we design photocatalysts with supramolecular recognition groups that bind, in the ground state, to the desired reagent. As such, we prevent excited state quenching by other reagents leading to a higher chemoselectivity. In addition, by the addition of, for example, a basic moiety in the recognition group, we can force proton-coupled electron transfer, which renders the back-electron transfer unviable. As such, we prevent charge recombination, which in turn increases the quantum yield for the desired reactivity.
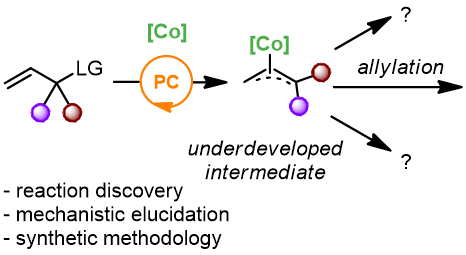
Metallaphotoredox Catalysis for Organic Synthesis
Metallaphotoredox catalysis is a recently developed strategy generally employed for the construction of carbon-carbon bonds using mild catalytic conditions. Although most of the research up to now has been done using a combination of photoredox catalysis and nickel or copper catalysts, cobalt has recently shown to possess unique reactivity. Therefore, the combination of photoredox catalysis and cobalt shows great promise for the development of novel reactions. We are currently especially interested in the production of cobalt-allyl complexes and their properties in following carbon-carbon bond formation. In addition, because of the novelty of these reactions, we are interested in developing a deep understanding of the reaction mechanisms in order to make metallaphotoredox catalysis a general tool for the organic chemist.